Which Designs Play to the Strength of Each Protocol?
The natural question, after you have decided that network design plays into the suitability of the protocol (you have seen this to be the case for convergence speed, but the same is also true of any other factor you might consider for a given routing protocol, including management, troubleshooting, configuration, and so on) is this:
- What sorts of network designs play into the strengths of any given routing protocol?
This is not an easy question to answer because of the numerous ways to design a network that works. Two- and three-layer network designs, switched cores versus routed cores, switched user access versus routed user access—the design possibilities appear to be endless. To try to put a rope around this problem, the sections that follow examine only a few common topological elements to illustrate how to analyze a specific topology and design and try to determine how a routing protocol will react when running on it.
The specific types of network topologies considered here are as follows:
- Hub-and-spoke designs
- Full mesh designs
- Highly redundant designs
After you consider each of these specific topology elements, you learn the general concepts of hierarchical network design and how each protocol plays against them.
Hub-and-Spoke Topologies
Hub-and-spoke network designs tend to be simple in theory and much harder in implementation. Scaling tends to be the big problem for hub-and-spoke topologies. The primary focus here is the capability of a routing protocol to maintain a multitude of routing neighbors and to converge to massive network events in an acceptable amount of time. Assume, throughout this section, that you are always dealing with dual-homed hub-and-spoke networks, as Figure G-2 illustrates.

Figure G-2 Dual-Homed Hub-and-Spoke Network
Start by considering the following simple question:
- How many spokes or remote routers does it take to really start stressing any routing protocol that is running over a hub-and-spoke network design?
The answer to this question always depends on various factors, including link speed and stability, router processing speed and packet switching speeds, and other factors. However, general experience shows that a high-speed router (in terms of processing power) with reasonably good design supports at least 100 remote sites with any modern routing protocol.
When considering network designs in which hundreds of remote sites are available, however, you need to use special techniques with each protocol to scale the number of remote sites attached to a single pair of hub routers. Look at each protocol to see what types of problems you might encounter and what types of tools are available to resolve those problems:
- OSPF floods topology information to each router within an area and summaries of reachability information into the area. You can place all the remote site routers into one or more OSPF stub areas, which cuts down on the amount of information flooded out to each remote site. Any change on a remote site is still flooded to every other remote site within the same area. For that reason, the design becomes a tradeoff between the number of areas that you want to manage and that the hub routers support and the amount of information that you can flood through the low-speed links connecting the remote stub sites.
- IS-IS also floods information to each router within an area. It does not, by default, flood information from the core of the network (the L2 routing domain) into each area. Again, you still face the tradeoff of how many level 1 routing domains you want to support at the hub routers versus how much information you can flood toward each remote router.
- The primary factor in determining scaling and convergence time in an EIGRP hub-and-spoke network is the number of queries the hub router needs to generate or process when the network changes, and the number of updates the hub router needs to generate toward the remote. Normally, if a hub loses several routes, for instance, it needs to generate queries for each of those routes to each of the remote sites. The remote sites then query the other hub router, which must process and reply to each of the queries. If the number of routes is high, this can be a processor- and memory-intensive task, causing the network to converge slowly, especially if the links between the remote sites and the hub routers are low speed. In this situation, you can summarize routers at the core toward the remote routers and block the routing information transmitted up toward the core routers. You can also cut down on the query range into the hub-and-spoke network dramatically. EIGRP, however, also provides a special operational mode for the remote sites; you can configure the remote sites as stubs, which indicates to the hub routers that the remote sites are never used for transiting traffic. If the remote sites are configured as stub routers, the hub router never queries them for lost routes, and the scaling properties change dramatically.
EIGRP, in theory, scales much better in a hub-and-spoke topology—and this is true in real networks, too. You often find EIGRP hub-and-spoke networks that have more than 500 remote sites attached to a pair of hub routers, over low bandwidth links, in the wild. In contrast, you tend to see OSPF and IS-IS hub-and-spoke networks top out at around 200 remote sites, even if higher bandwidth links are involved.
Full Mesh Topologies
Full mesh topologies are a less common design element in networks, but they are worth considering because the scaling properties of a routing protocol in a full mesh design indicate, to some degree, the scaling properties of the same protocol in a partial mesh design. You can think of a full mesh topology as a special case of a partial mesh topology. Again, look at the challenges and tools that are available for each protocol. Use the network illustrated in Figure G-3 throughout this discussion.
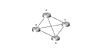
Figure G-3 Full Mesh Network
- Each OSPF router sends topology information to each adjacent neighbor within an area (flooding domain). If Router A receives a new link-state advertisement (LSA), Router D receives three copies of this new LSA: one from Router A, one from Router B, and one from Router C. The Cisco IOS Software implementation of OSPF does have an option to control the flooding through a full mesh network, using the database filter-out command.
- IS-IS is similar to OSPF; each router sends topology information to each adjacent neighbor. Cisco IOS Software enables you to control flooding through mesh groups.
- Each router in an EIGRP network sends each of the routes it is using to forward traffic to each neighbor. In this network, Router D is going to receive three copies of any new routing information that Router A receives, one copy from Router A, one from Router B, and one from Router C. These three copies of the routing information might be the same, but they indicate reachability through three different next hops (or neighbors). Reducing the information propagated through the mesh is difficult, at best. You can filter these routing updates through some paths within the mesh to decrease the amount of information flooded through the mesh, but that also reduces the number of paths usable through the mesh for any specific destination.
OSPF and IS-IS flood extra information through a mesh topology by default, but you can use tools to reduce the amount of flooding in highly meshed topologies. EIGRP sends updates through each router in the mesh, but it is difficult to reduce the number of these updates unless you want to decrease the number of paths that the network actually uses through the mesh.
In the real world, OSPF and IS-IS scale better in highly meshed environments, especially if you implement flooding reduction techniques. This is a matter of scale, of course; networks that have a mesh network of 20 or 30 routers work fine with any of the three routing protocols. However, when the mesh starts surpassing this number of routers, the special techniques that OSPF and IS-IS offer to scale further can make a difference.
Interaction with Hierarchical Designs
Traditional network design is based on layers, either two or three, that abstract the network details into "black boxes" and divide functionality vertically through the network to make management and design easier:
- The two-layer model has aggregation and core layers, or areas, within the network.
- The three-layer model has access, distribution, and core layers.
How do these layered network designs interact with each protocol? Consider each protocol in turn.
OSPF splits flooding domains into areas that are separated by ABRs. Because every router within an area must share the same link-state database to calculate loop-free paths through the network, the only place that route aggregation can be performed is at an ABR. ABRs actually aggregate two types of information:
- Information about the topology of an area that is hidden from other areas at these border edges
- Aggregation of reachability information that can be configured at these border edges
This combination of route aggregation points and flooding domain boundaries in the network implies several things:
- In all three-layer network designs with OSPF, you should place the ABR in the distribution layer of the network.
- In all two-layer network designs with OSPF, you should place the ABR at the aggregation to core layer edge of the network.
- The most aggregation points that you can cross when passing from one edge of the network to the opposite edge of the network is two.
These topological limitations might not be major in smaller networks, but in networks that have thousands of routers, they could impose severe restrictions on the network design. Network designers and operators normally break up OSPF networks at this size into multiple administrative domains, connecting the separate domains through BGP or some other mechanism.
IS-IS is similar to OSPF in its restrictions, except that IS-IS allows the core and outlying flooding domains to overlap. This introduces a degree of flexibility that OSPF does not provide, but you can still only aggregate routing information at the edges where two flooding domain meet, and you cannot build more than two levels of routing into the network.
EIGRP, as a distance vector protocol, does not divide the concepts of topology summarization and routing aggregation; topology beyond one hop away is hidden by the natural operation of the protocol. Figure G-4 illustrates the conceptual difference among EIGRP, OSPF/IS-IS, and RIP in terms of topology information propagated through the network.
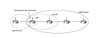
Figure G-4 Topological Awareness in Routing Protocols
If you examine the scope through which routing information is transmitted (or known) within a network, you find the following:
- The Bellman-Ford algorithm, used by the Routing Information Protocol (RIP) and the Interior Gateway Routing Protocol (IGRP), uses only information about the local cost to reach a given destination. If Router B is running RIP, it considers only the total cost of the path to reach a destination at Router E when deciding on the best (loop-free) path.
- Diffusing Update Algorithm (DUAL), used by EIGRP, considers the local cost to reach a given destination and the cost of each neighbor to reach the same destination when calculating which available paths are loop free. EIGRP uses an awareness of the topology that is one hop away from the calculating router.
- OSPF and IS-IS, which are link-state protocols, do not use information about the metrics of a neighbor; rather, they count on being aware of the entire topology when calculating a loop-free path. At a flooding domain border, OSPF and IS-IS act much like distance vector protocols. Router A does not know about the topology behind Router B; it only knows the cost of Router B to reach destinations that are attached to Router E.
Because topology information is hidden in the natural processing of EIGRP routing updates, EIGRP is not restricted in where it can aggregate routing information within the network. This provides a great deal of flexibility to network designers who are running EIGRP. Multiple layers of aggregation can be configured in the network. This means that moving from one edge of the network to the opposite edge of the network could mean encountering many more than two aggregation points.
The practical result of the EIGRP capability to aggregate routing information anywhere in the network is that many existing large-scale (2000 router and larger) networks run within a single EIGRP process or administrative domain. The feasibility of building networks this large is based on the capability to use route aggregation to divide the network into multiple layers, or sections, each acting fairly independently of the other. Although it is possible to build an OSPF or IS-IS network this large, designing and managing this network is more difficult because of the restrictions that link-state protocols place on aggregation points.
In general, up to some relative size, the protocols are relatively equal in their capability to work with hierarchical network designs. OSPF and IS-IS tend to be less flexible about where route aggregation can be placed in the network, making it more difficult, in some situations, to fit the network design and the protocol design together. EIGRP excels at fitting into hierarchical network design.
Topological Rules of Thumb
After examining these various network topologies and how each routing protocol tends to react, you can see that when a network does not reach the edge of a specific protocol capability on any given topology, any of the routing protocols is fine. If your network has a specific predominant topology type, however, such as large-scale hub-and-spoke or large-scale full mesh topologies, choosing a protocol to fit those topologies makes sense. You can always compromise in complex areas of your network design by making effective and stable topological design areas in which the routing protocol is really stretched to the edge of its capabilities.